The issue of air quality management has gained significant attention globally due to the increasing levels of air pollution and its detrimental effects on human health and the environment. Gas sensors, as critical tools in air quality monitoring, have undergone significant advancements in recent years. This article delves into the emerging gas sensor technologies and their contributions to improving air quality management. It discusses the various types of gas sensors, their principles of operation, applications in air quality monitoring, challenges faced, and future prospects.
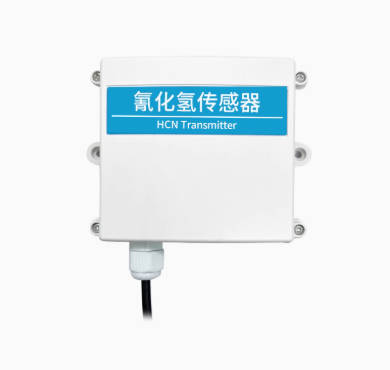
Keywords: gas sensors, air quality management, emerging technologies, environmental monitoring, health impacts
Introduction
Air pollution is a complex environmental challenge that affects urban and rural areas alike. It encompasses a wide range of pollutants, including particulate matter, nitrogen dioxide (NO₂), sulfur dioxide (SO₂), carbon monoxide (CO), ozone (O₃), and volatile organic compounds (VOCs). These pollutants can originate from various sources such as industrial emissions, vehicular exhaust, residential heating, and agricultural activities. Long-term exposure to air pollution has been linked to various health issues, including respiratory diseases, cardiovascular problems, and even cancer.
Effective air quality management requires accurate and timely monitoring of air pollutants. Gas sensors play a pivotal role in this process by detecting the presence and concentration of specific gases in the atmosphere. Over the past few decades, significant advancements have been made in gas sensor technology, leading to the development of more sensitive, selective, and reliable sensors.
Types and Principles of Gas Sensors
Metal Oxide Semiconductor (MOS) Gas Sensors
MOS gas sensors are among the most widely used gas sensors due to their low cost, small size, and ease of integration. They operate based on the change in electrical conductivity of metal oxide semiconductors upon exposure to target gases. When a gas molecule interacts with the surface of the metal oxide, it alters the charge carrier concentration, leading to a change in resistance that can be measured as an electrical signal.
MOS gas sensors are particularly sensitive to reducing gases such as CO and VOCs. However, they can also detect oxidizing gases like NO₂ and O₃ with proper modification. One of the key challenges with MOS gas sensors is their cross-sensitivity to multiple gases, which can lead to false positives or negatives.
Electrochemical Gas Sensors
Electrochemical gas sensors measure the concentration of gases by converting chemical energy into electrical energy through an electrochemical reaction. They are highly selective and sensitive to specific gases, such as NO₂, SO₂, CO, and O₃. Electrochemical gas sensors consist of a working electrode, a counter electrode, and a reference electrode, which are immersed in an electrolyte solution. When the target gas diffuses into the electrolyte, it undergoes an oxidation or reduction reaction at the working electrode, resulting in a current flow that is proportional to the gas concentration.
Optical Gas Sensors
Optical gas sensors utilize the interaction between light and gas molecules to detect the presence of specific gases. They can be classified into several types, including infrared (IR) absorption sensors, photoacoustic sensors, and chemiluminescence sensors.
IR absorption sensors measure the absorption of IR radiation by gas molecules at specific wavelengths. This absorption is directly related to the concentration of the gas. Photoacoustic sensors detect the sound waves generated when a gas absorbs modulated light and undergoes thermal expansion and contraction. Chemiluminescence sensors measure the light emitted when certain gases react chemically with other substances.
Other Emerging Gas Sensor Technologies
In addition to the aforementioned technologies, several other emerging gas sensor technologies are gaining attention. These include gas sensors based on microelectromechanical systems (MEMS), graphene, and conducting polymers.
MEMS gas sensors integrate mechanical and electrical components on a single chip, enabling high sensitivity and selectivity. Graphene-based gas sensors exploit the unique electronic properties of graphene to detect gases with high precision. Conducting polymer gas sensors utilize the conductivity changes in conducting polymers upon exposure to target gases.
Applications in Air Quality Monitoring
Urban Air Quality Monitoring
Urban air quality monitoring is crucial for identifying pollution hotspots, tracking pollution trends, and evaluating the effectiveness of pollution control measures. Gas sensors are deployed in various locations within cities, including roadside stations, industrial areas, and residential neighborhoods. They provide real-time data on the concentration of pollutants, enabling authorities to take prompt action when pollution levels exceed safe limits.
For instance, the city of London has deployed a network of low-cost gas sensors as part of its "Breathe London" project. These sensors monitor NO₂ levels and provide real-time data to the public through a mobile application. This initiative has helped raise awareness about air pollution and encouraged community participation in air quality improvement efforts.
Industrial Emissions Monitoring
Industrial emissions are a significant source of air pollution. Gas sensors are used to monitor emissions from factories, power plants, and other industrial facilities. They help ensure compliance with environmental regulations and facilitate the implementation of pollution control measures.
In the steel industry, for example, gas sensors are used to monitor emissions of CO, NO₂, and SO₂. By continuously monitoring these pollutants, factories can optimize their production processes to minimize emissions and reduce their environmental footprint.
Indoor Air Quality Monitoring
Indoor air quality is equally important as outdoor air quality, as people spend a significant portion of their time indoors. Gas sensors are used to monitor indoor air pollutants such as VOCs, CO₂, and formaldehyde. They help identify sources of pollution, such as paint, cleaning products, and building materials, and enable the implementation of effective ventilation and air purification strategies.
Smart buildings, for instance, integrate gas sensors with other IoT devices to monitor and control indoor air quality. By automatically adjusting ventilation rates and activating air purifiers when pollutant levels rise, these buildings can ensure a healthy and comfortable indoor environment for occupants.
Challenges and Limitations
Selectivity and Cross-Sensitivity
One of the major challenges in gas sensor technology is achieving high selectivity towards target gases while minimizing cross-sensitivity to other gases. Cross-sensitivity can lead to false positives or negatives, compromising the accuracy of air quality data.
Researchers are continuously developing new materials and sensor architectures to improve selectivity. For example, the use of nanomaterials, such as metal oxide nanoparticles and graphene, has shown promising results in enhancing the selectivity of gas sensors.
Long-Term Stability and Calibration
Gas sensors can experience drift over time due to factors such as aging of sensor materials, contamination, and changes in environmental conditions. This drift can affect the accuracy and reliability of sensor readings.
Regular calibration of gas sensors is essential to ensure accurate data. However, calibration can be time-consuming and costly, particularly for large-scale sensor networks. The development of self-calibrating gas sensors and the use of machine learning algorithms for drift correction are potential solutions to this challenge.
Cost and Scalability
While gas sensor technology has made significant progress in terms of performance, the cost of sensors remains a barrier to widespread adoption. High-performance gas sensors, such as those based on MEMS and graphene, can be expensive to manufacture.
Scaling up sensor networks to cover large areas requires a significant investment in infrastructure and maintenance. The development of low-cost, robust, and easy-to-deploy gas sensors is crucial for realizing the full potential of gas sensor technology in air quality management.
Future Prospects
Integration with IoT and Big Data Analytics
The integration of gas sensors with IoT technology and big data analytics is poised to revolutionize air quality management. By connecting gas sensors to the internet, real-time data can be collected, analyzed, and shared across different platforms.
Big data analytics can be used to identify pollution patterns, predict pollution events, and evaluate the effectiveness of pollution control measures. For example, machine learning algorithms can be trained to predict air quality based on historical data and real-time sensor readings. This information can be used to inform policy decisions and guide public health interventions.
Development of Novel Sensor Materials and Architectures
The ongoing research in nanotechnology and materials science is expected to lead to the development of novel sensor materials and architectures with improved performance. For instance, the use of two-dimensional materials such as graphene and transition metal dichalcogenides (TMDs) has shown promise in enhancing the sensitivity and selectivity of gas sensors.
The development of flexible and wearable gas sensors is also gaining momentum. These sensors can be integrated into clothing, smartwatches, and other wearable devices, enabling continuous monitoring of personal exposure to air pollutants.
Policy and Regulatory Support
Government policies and regulations play a crucial role in promoting the adoption of gas sensor technology in air quality management. Policies that encourage the development and deployment of low-cost, high-performance gas sensors can accelerate technological progress and improve air quality.
Regulatory frameworks that mandate the monitoring of specific pollutants and set stringent emission limits can also drive the demand for gas sensors. By working together with industry stakeholders, governments can facilitate the development and deployment of gas sensor technology to address air pollution challenges.
Conclusion
Emerging gas sensor technologies are playing an increasingly important role in improving air quality management. By providing real-time data on the concentration of pollutants, these sensors enable authorities to take prompt action to mitigate air pollution and protect public health.
While challenges such as selectivity, long-term stability, and cost remain, ongoing research and technological advancements are expected to address these issues. The integration of gas sensors with IoT technology and big data analytics, the development of novel sensor materials and architectures, and policy and regulatory support will further propel the growth of gas sensor technology in air quality management.