The global agricultural sector stands at a critical juncture, facing escalating pressures from climate change, population growth, and resource depletion. Droughts, erratic rainfall, and soil degradation threaten food security, while conventional farming practices—characterized by over-irrigation, blanket fertilization, and reactive decision-making—exacerbate environmental degradation. To meet the dual challenge of feeding a projected 9.7 billion people by 2050 while preserving ecosystems, farmers must adopt sustainable, data-driven practices.
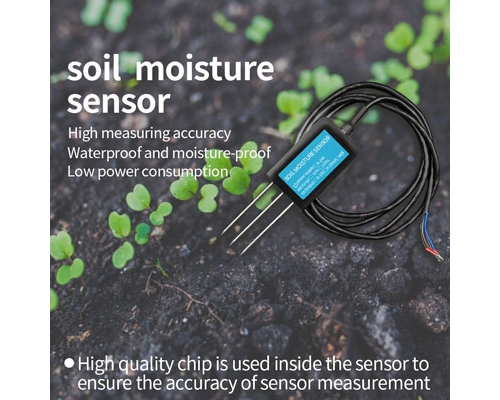
At the forefront of this transformation are IoT-enabled soil sensors, which provide real-time, spatially granular insights into soil health. These devices, deployed in networks across fields, enable farmers to monitor drought conditions, map soil fertility, optimize resource use, and adopt regenerative practices. This article explores the multifaceted role of IoT soil sensors in sustainable farming, their technical underpinnings, real-world applications, and their potential to redefine agriculture in the 21st century.
1. The Imperative for Sustainable Agriculture: Why Soil Sensors Matter
Conventional farming relies on static management plans—irrigating fields uniformly, applying fertilizers at fixed rates, and planting without regard for soil variability. This approach leads to:
Water Waste: Over 70% of global freshwater withdrawals are used for agriculture, with up to 50% lost to inefficiencies.
Nutrient Pollution: Excess fertilizers run off into waterways, causing algal blooms and dead zones.
Soil Degradation: Poor irrigation and tillage practices degrade soil structure, reducing its carbon-sequestering capacity.
IoT soil sensors address these issues by enabling precision agriculture, where inputs are tailored to spatial and temporal variability. By providing actionable data on soil moisture, nutrients, temperature, and salinity, these sensors empower farmers to:
Detect droughts early and adjust irrigation.
Map soil fertility to apply fertilizers only where needed.
Reduce chemical runoff and greenhouse gas emissions.
Enhance soil health for long-term resilience.
2. How IoT Soil Sensors Work: Technology and Integration
IoT soil sensors are compact, wireless devices that measure key soil parameters and transmit data via low-power networks. Their core components include:
A. Sensing Mechanisms
Moisture: Capacitive sensors (e.g., Decagon ECH₂O) or time-domain reflectometry (TDR) probes measure volumetric water content.
Nutrients: Ion-selective electrodes (ISEs) detect nitrate (NO₃⁻), ammonium (NH₄⁺), phosphate (PO₄³⁻), and potassium (K⁺). Optical sensors (e.g., NIR spectroscopy) estimate organic matter and CEC.
Temperature and Electrical Conductivity (EC): Thermistors and EC probes monitor root-zone conditions, which influence nutrient uptake and microbial activity.
pH and Salinity: Real-time pH sensors (e.g., Atlas Scientific) and EC meters assess soil acidity and salt accumulation.
B. Connectivity and Data Transmission
Sensors communicate via:
LPWANs (Low-Power Wide-Area Networks): LoRaWAN, Sigfox, or NB-IoT enable long-range, battery-efficient data transmission.
Cellular and Satellite: For remote areas without terrestrial coverage (e.g., Australian outback farms).
Mesh Networks: Devices relay data to gateways, reducing infrastructure costs (e.g., Zigbee or Z-Wave).
C. Cloud Platforms and Analytics
Raw sensor data is processed in the cloud using:
Machine Learning (ML): Algorithms predict soil moisture trends, nutrient dynamics, or disease risks.
GIS Mapping: Spatial visualizations highlight variability across fields (e.g., "hotspots" of low phosphorus).
Decision Support Systems (DSS): Platforms like CropX or Farmers Edge generate irrigation/fertilization prescriptions.
3. Core Applications of IoT Soil Sensors in Sustainable Farming
A. Drought Monitoring and Irrigation Optimization
Droughts cost the global economy over $124 billion annually, yet 40% of irrigation water is wasted due to inefficiencies. IoT sensors enable:
Real-Time Alerts: Farmers receive SMS/email notifications when soil moisture drops below critical thresholds.
Variable-Rate Irrigation (VRI): Sensors guide center-pivot systems to apply water only to dry zones (e.g., a California almond orchard reduced water use by 35% using VRI).
Evapotranspiration (ET) Modeling: Combining soil moisture data with weather forecasts to schedule irrigation precisely (e.g., Israel’s Netafim systems cut water use by 50%).
B. Fertility Mapping and Precision Fertilization
Over-fertilization contributes to eutrophication and nitrous oxide emissions (a greenhouse gas 300x more potent than CO₂). IoT sensors facilitate:
Zone-Specific Nutrient Management: Sensors identify nutrient-deficient areas, allowing targeted applications (e.g., a corn farmer in Iowa saved $80/acre on fertilizer).
Slow-Release Fertilizer Timing: By tracking nutrient release rates in real-time, sensors optimize timing for maximum uptake (e.g., urea-coated sensors in Brazil).
Organic Matter Tracking: Regular soil carbon measurements help farmers adopt carbon farming practices (e.g., cover cropping) to boost fertility.
C. Salinity and pH Management for Soil Health
Soil salinization affects 20% of irrigated lands globally, reducing yields by 50%. IoT sensors combat this by:
Salinity Hotspot Detection: Early warnings allow leaching or gypsum applications to restore soil structure (e.g., Pakistan’s Indus Basin farmers).
pH-Based Lime Application: Sensors guide lime dosing to correct acidity, improving nutrient availability (e.g., tea plantations in Sri Lanka).
Crop-Specific Recommendations: AI platforms cross-reference soil data with crop tolerance thresholds (e.g., avocados prefer pH 6–6.5).
D. Disease and Stress Prediction
Stressed plants are more susceptible to pests and pathogens. IoT sensors detect early warning signs by:
Root Health Monitoring: High EC or low moisture can indicate root rot; sensors trigger biocontrol agents (e.g., trichoderma) or aeration.
Microclimate Adjustments: In greenhouses, soil and air sensors coordinate to prevent fungal outbreaks (e.g., Dutch tomato growers reduce fungicide use by 40%).
4. Real-World Success Stories: Scaling Sustainability
A. Case Study 1: Wheat Farming in Australia’s Murray-Darling Basin
A 10,000-hectare farm deployed 200 IoT soil sensors to combat drought. By:
Mapping soil moisture variability,
Adjusting irrigation zones dynamically, and
Using ML to forecast water needs,
the farm reduced water use by 28% while increasing wheat protein content (a premium market trait) by 12%.
B. Case Study 2: Coffee Plantations in Colombia
Smallholder farmers adopted low-cost soil moisture and pH sensors linked to mobile apps. By:
Avoiding over-irrigation during rainy seasons,
Correcting acidic soils with targeted lime, and
Accessing microloans based on soil health data,
they doubled yields and reduced fertilizer costs by 35%.
C. Case Study 3: Rice Paddies in India’s Punjab Region
A cooperative installed solar-powered soil nutrient sensors to combat groundwater depletion. By:
Applying nitrogen only to deficient zones,
Adopting alternate wetting-drying (AWD) irrigation, and
Monitoring methane emissions (via gas sensors),
they cut water use by 40% and methane by 22%, qualifying for carbon credits.
5. Economic and Environmental Trade-offs: Balancing Profit and Planet
While IoT soil sensors offer clear benefits, their adoption involves trade-offs:
A. Cost-Benefit Analysis
Pros:
Input savings (water, fertilizer) typically offset sensor costs within 2–3 years.
Yield premiums for sustainably grown crops (e.g., EU’s eco-labels).
Cons:
B. Environmental Risks and Mitigations